Pho’liage ®
December 2019
Biomimetism
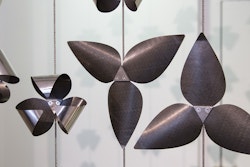
This paper introduces an innovative façade design which bears the project name PHO’LIAGE®, whose inspiration was drawn from flower-like opening and closure mechanisms observed in the plant kingdom. AB_Lab’s research has been focused on the development of efficient biomimetic façade components which react to variations in the sun’s radiation intensity. The employment of smart materials in designs which mimic thermonastic and photonastic behaviour in plants helps us establish a promising proof of concept which could lead to increased energy efficiency in buildings.
We present a summary of the theoretical and practical models that informed the evolution of the concept, outlining protocols used and offering analysis of results obtained thus far. Our design aims to overcome technical issues commonly involved in designing and manufacturing curved façade components, including assessments of aperture ratio efficiency and outdoor corrosion sensitivity of the actuator (made from thermostatic bimetal or TBM). Both computer-aided design modelling and physical testing were used to improve the design. Further innovations of the solar shading device are introduced in the form our most recent prototype which features photovoltaic (PV) sub-elements. The production of renewable energy thanks to the PV cells differentiates this dynamic shading system from any other existing solution.
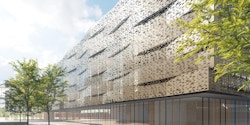
1. Introduction
ES-SO studies confirm that more than one third of Europe’s total primary energy is consumed by the building construction sector and building management field [1]. Building façades are responsible for more than 40% of the total energy loss in the winter, as well as over-heating in the summer [2], making the employment of air conditioning systems inevitable in the provision of adequate internal comfort for users and building occupants.
Space cooling systems (SC) account for an important part of the average European Union’s household’s air conditioning energy consumption (about 5%), and are particularly present in the service sector (approximately 13%) [3] [4]. The European space cooling market is characterised by a huge potential for growth, with the percentage of the surface areas in the service sector being almost ten times higher (30%) than in the residential buildings (4%) [5] [6] [7].
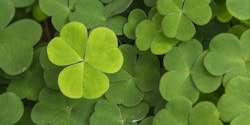
Given the expressed desire by the European markets for increased comfort standards, surface cooling applications are expected to increase, especially within the residential sector [8]. Further possible reasons for the future rise of SC application in Europe are the global warming and modern architectural designs with larger glazing areas [9]. This anticipated rise in cooling energy consumption creates a self-nurturing negative feedback loop and amplifies climate change significantly.
While the passive housing concept is often achieved by using high performance insulation materials in façades, the overuse of insulators can lead to an increased loads on ventilation and humidity regulation systems, which together amount to additional costs and significant space losses. “Ventilation units consume more than 2% of all electricity in the EU and are amongst the biggest consumers of indoor electricity, after heating, cooling, and lighting” [10].
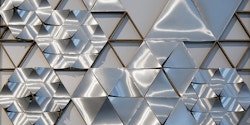
With accurate design of façade details and efficient solar shading systems, office buildings in Europe could plausibly work without high energy-consuming cooling systems. By reducing the use of ventilation systems through alternative solutions, Europeans could save “approximately 1300 PJ in energy use each year by 2025. This is equivalent to the annual gross energy consumption of Austria or Greece” [10]. Following these premises, in EU, the energy consumption of building construction could potentially be reduced by 10% for oil consumption (approx. 41 m tons) and around 111 m tons for CO2 production per year [1].
The use of adaptive shading systems with integrated PVs could contribute to reduce the energy demand of building’s and solve the challenging climate change and pollution problems. Thanks to the dynamic natural light barrier of our kinetic device, PHO’LIAGE® may reduce the heating energy demand in wintertime while in summer and the integrated PV cells protect the façade from overheating by transforming the sun energy surplus into usable green electricity.
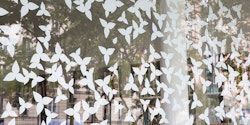
2. A review of existing state-of-the-art solutions
Our team used a Top-Down approach in order analyse existing dynamic façade systems, followed by a Bottom Up approach in the development of the functional components of the PHO’LIAGE TBM-PV shading device. Most existing kinetic shading devices which follow similar paths to our own are still under R&D improvement. Not all dynamic shading systems were taken into consideration, only the most seemingly relevant to our solution were analyzed or benchmarked [11].
The Al Bahr Towers façade [12] (Abu Dhabi [UAE], 2009-2013) is a well-known reference in kinetic biomimetic sun-shading solutions. The motorized supporting structures and the necessity of sensors, processors and software are costly financially and energetically. In other terms, this system may not bring an important large-scale and eco-responsible solution to the EU long-term environmental challenges due to the high energy input and maintenance fees [13]. After studying and trying to improve other innovative solutions such as the kinetic façade designed for the Institut du Monde Arabe [14] [15] (Paris, 1987), inspired by the traditional mashrabiya pattern [16], Flectofold [17] (Stuttgart [Germany], 2017) and Flectofin® [18] (Stuttgart [Germany], 2011), our R&D team chose not consider those concepts as promising enough in terms of large-scale impact. The need of activation energy input, complicated industrialisation process or the exceeding maintenance cost were the main counterarguments.
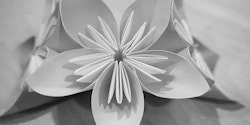
Andrew Payne’s AirFlow(er) [19] (Cambridge [USA, MA], 2007-2018) is designed for ventilation purposes, with intrinsic control (sensing and actuation are embedded in the material) bypassing the need for high-tech equipment. The activation is mechanical but is combined with the use of Shape Memory Alloy (SMA) wire, which is more commonly known as another thermosensitive smart material. This material has memory-shaped activation properties similar to the TBM that we use in the PHO’LIAGE® concept. The difference lies in fact that the limited contraction of the Nitinol® SMA wire significantly reduces the extent of the AirFlow(er) panels’ movement, when compared to PHO’LIAGE® aperture ratio actual performance.
Doris Sung’s Bloom [20] pavilion (Los Angeles [USA, CA], 2012) shows the use of TBM for the first time in an exterior architectural application. The installation acts as a sun tracking instrument indexing time and temperature with specifically cut panels acting together as an environmentally responsive form. The project is designed for peak performance on the spring equinox, and is maintained by a complex digital software in comparison to the PHO’LIAGE® self-maintaining passive technology, activated only by the TBM material properties and the biomimetic design, managed fully by the sun radiation energy input.
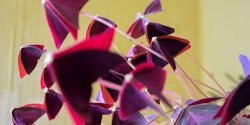
Achim Menges’s HygroSkin [21] (Orléans [France], 2013) project led us to investigate the behaviour of nastic structures in plants at a biomechanical level and led to the team performing multiple phases of biomimetic pattern abstraction. We do not consider HygroSkin as a concurrent solution as the latter requires humidity coefficient change as a physical actuator, which we do not consider pertinent when developing a sunlight or heat stimulated shading device. Design methods: the “Top-Down-Process” inspired by biomimetics.
The research team chose a “Top-Down” process, often used in biomimetics as a catalyst in the development of bio-inspired solutions. Charles Darwin was among the first to study how plants change shapes in his seminal work, The Power of Movement in Plants (1881). Darwin’s study of the sleep movements of cotyledons and leaves, characterised by a diurnal/open position and a folded/nocturnal position [22] [23] (See Figure 2 and 3), were of significance in our search for appropriate physical models. Other scientists such as Ingo Burgert and Peter Fratzl reviewed various opening mechanisms and demonstrated how useful their translation into technical devices could it be [24].
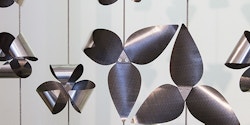
2.1 Finding ground elements: From continuous to discrete elements
Initial concepts explored by the team involved the direct use of thermo-bimetal bands with incisions cut parametrically into the material. This approach was chosen as appropriately frugal given the cost of TBM materials and the difficulty of tooling the bands. It also effectively employed the factory curved shape memory, as the band of bi-metal were stretched flat from their initial factory roll configuration, and the incision would ‘free’ the bands according to the shape of each cut, allowing them to take up their initial memory shape. As the curved shapes heat up in the sun, they go back to ‘lay flat’ position and thus close the gaps made by the incisions, protecting the façade from solar gain. (Figure 1).
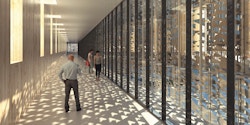
Practical tests with both thermo bi-plastics and thermo bi-metals led the team to focus on triangular shapes which best expressed curving responses to heat changes as well as rigidity, anticipating the needs for the facades elements to be positioned on the exterior of the build envelope in order to be effective, all the while subjected to wind loads and pollution.
The form finding process followed several steps. Based on the studies of the existing solutions together with the available literature [15] [25], the team conducted a detailed feasibility studies using manufacturer’s data for TBM material in use. A reference for thermostatic bimetal produced by Engineered Material Solutions® [26] was selected for its temperature activation range and relative mechanical resilience vs action span. A theoretical model was then created using the finite element method (FEM). In combination with wind-load calculations and the applied thermostatic theory of Antoine Yvon Villarceau [27], the first 3D parametric models were built on Rhinoceros® and Grasshopper®. Those 3D models helped to visualise and calculate the open / closed optimal ratio induced by a temperature change, before engaging into the physical testing as next step of the R&D process (Figure 2).

The team then tested prototype of assembled individual triangular ‘petals’ which made more efficient use of the TBM material. The resulting ‘flowers’ or petal clusters are connected by stainless steel cables to be mounted, once again, on the exterior of the façade. This configuration led to more specific studies on the detailing of attachment components given the need to both hold the TBM petals in place, all the while allowing for their movement when required.
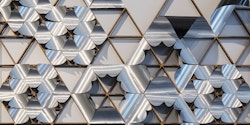
2.2 Optimising shapes and design
Flower petal movements in the living world are mostly induced by a difference in growth rate on each side of the petal. In species that open in the morning primarily as a response to temperature, the inner surface of the petal rapidly expands when the temperature raises due to the release of phytohormone metabolites, notably absent on the outer surface. Cooling results in a faster growth on the outer surface. Such movements are usually described as nastic, “or direction-independent, reversible orientational changes in response to direction-independent stimuli” [28], in plants at a biomechanical level. Study of these phenomena by the research team led to the development of several bio-inspired TBM-made prototype propositions (Table 1). The change in the TBM curving shape is memory-shaped which makes possible the long-term use of our optimised design without any maintenance.
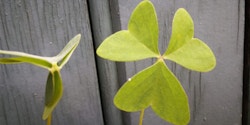
Based on a combination of different shapes and numbers for petals, the team tested various configurations [29] taking into account the physical properties of the material which were not available through the catalogue literature. This modelling was especially aimed at minimising the loss of materials during the punch press industrial process, as well as locating the critical actuating surfaces in areas when sunlight masking would be enhanced or avoided.
The following key elements came to the fore in the design of subsequent prototypes:
- Optimizing the functional area of the TBM Actuator and reducing the masking effect
- Optimizing the open/folded ratio.
- Reducing the sound made by material distortion (Field Level in dB) of the bimetal elements when heated and cooled.
As result of this study, the perforated design of the V2 thermostatic bimetal element was chosen as the best choice regarding the specific application as actuator of the shading device (See Figure 6 and 7). Lab test results supported our trilobal petal-like TBM actuator design, which reduced material waste, increased the open/close ratio efficiency, reduced sound emissions during the activation process, and showed slower ‘closing time’ during the cooling process leading to increased solar shading performance.
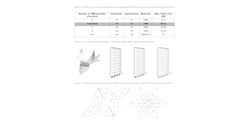
3. Prototyping: Real life models and materiality
Once the ground components were defined in terms of material specification and general shape, it was possible to create a larger mock-up model (See Figure 8).
The research team then focused on corrosion protection, all the while maintaining the intention to optimize the duration and reactivity of the petal curving dynamics. Through combining a series of decorative coatings (Cr-VI free) that also act as anti-corrosion protection of the TBM smart material, the necessary flexibility related to the overall memory-shaped performance was maintained.
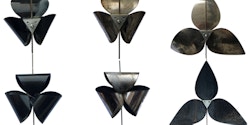
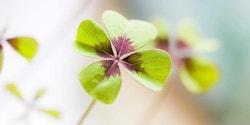
3.1 TBM actuator with integrated PV cells
Having produced several prototypes at different stages of the R&D, the team tested different assembly configurations of the TBM actuator and PV cells subcomponents in order to explore a cost-effective solution with the highest possible efficiency and reactivity. The team continues to work on this concept through theoretical models and lab tests. The renewable electricity production combined with the dynamic memory-shaped movement of the TBM actuator distinguish this patent pending technology from other existing solutions (See Figure 7 and 8).
As mentioned previously, the solar shading device works without motorization or maintenance needs in the timeframe of the warranty period. This unique passive kinetic device is activated and controlled fully by the solar radiation. The PV cells induce important heat loss during energy production cycles, mostly because of the Joule heating effect as process output. The positive interaction of both photo-sensitive (PV) and thermo-reactive (TBM) materials heat exchange is beneficial to the overall energy efficiency of the device resulting from the improvement of the actuator reactivity.
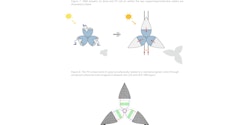
3.2 Increased efficiency despite the installation on a façade
Another benefit may be found in the tracking behavior which occurs through the interaction between TBM aperture memory-shaped movement and the PV cells, increasing significantly the kWh/year production when compared to a fixed PV alternative on a south-oriented façade. Dynamic movements of the shade-making device in response to the sunlight can thus increase PV production through better orientation.
Monocrystalline PV panels’ production cost has significantly decreased over recent years [30] [31], and the output efficiency is set to continue rising. We studied the PV cells’ integration into south-oriented façades in order to obtain an additional renewable energy production values and add the bonus of return on investment. We are aware that a vertical façade with fixed PV panels is less efficient that a PV roof installation due to the lower sun’s incidence angle in vertical applications, but having formulating a detailed feasibility study, our R&D team considers that is still worth going further with the release of the competitive PHO’LIAGE® kinetic façade technology whilst continuously improving it.
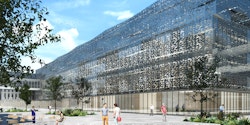
4. Discussion: the architectural scale
The team has now been developing this concept alongside their architectural projects and diversified research activities since 2011. Numerous experiments and engineering studies helped us to define an optimal shape for each subcomponent and guided us through the technology readiness levels (TRL), the intellectual property initiatives and, hopefully, product release. With an overall TRL8, we have successfully created an innovative proof of concept for a passive kinetic sun shading device for outdoor use, combining smart TBM memory-shaped materials with PV cells integrated functioning.
In 2022, PHO’LIAGE® will be implemented as a pilot project in the new headquarters of the International Agency for Research on Cancer (IARC) [34] [35] in Lyon France, a project for which ArtBuild are also lead architects.
Further research and prototyping are needed in order to improve the overall performance of the TBM-PV solar shading device. We believe that our work could inspire future visions about sustainable construction and create positive feedback in light of EU climate action goals.
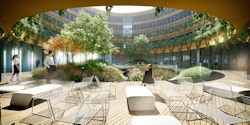
5. References
[1]
EUROPEAN SOLAR-SHADING ORGANIZATION, Energy Saving and CO2 Reduction Potential from Solar-Shading Systems and Shutters in the EU-25, 2006, retrieved from http://www.buildup.eu/sites/default/files/ESCORP-EU25_p2528.pdf.
[2]
M. De Groote, J. Volt, and F. Bean, Is Europe Ready for the Smart Buildings Revolution? – Mapping Smart-Readiness and Innovative Case Studies, Buildings Performance Institute Europe (BPIE), 2017, retrieved from http://bpie.eu/wp-content/uploads/2017/02/STATUS-REPORT-Is-Europe-ready_FINAL_LR.pdf.
[3]
EUROSTAT, “Electricity production, consumption and market overview: Net electricity generation, EU-28, 1990-2016”, July 2018, retrieved from https://ec.europa.eu/eurostat/statistics-explained/index.php/Electricity_production,_consumption_and_market_overview.
[4]
EUROSTAT, “Electricity and heat statistics: Gross electricity production by fuel, GWh, EU-28, 2000-2016 (nrg_105a)”, June 2018, retrieved from https://ec.europa.eu/eurostat/statistics-explained/index.php/Electricity_and_heat_statistics.
[5]
S. Werner, “European space cooling demands”, Energy, 2016, vol. 110, No. C, pp. 148–156.
[6]
ODYSSEE-MURE, “Household Energy Consumption by Energy in the EU”, retrieved from http://www.odyssee-mure.eu/publications/efficiency-by-sector/households/household-eu.pdf.
[7]
U. Persson, S. Werner, Stratego – Enhanced Heating & Cooling Plans: Quantifying the Heating and Cooling Demand in Europe: Work Package 2 / Background Report 4, Halmstad University, 2015, retrieved from http://stratego-project.eu/wp-...
[8]
S. Pezzutto, R. Fazeli, M. De Felice, W. Sparber, “Future development of the air-conditioning market in Europe: An outlook until 2020”, WIREs (Wiley Interdisciplinary Reviews): Energy and Environment, 2016, Vol. 5, No. 6, 649–669.
[9]
K. Shanks, E. Nezamifar, “Impacts of climate change on building cooling demands in the UAE”, Proceedings of the SB13 Dubai: Advancing the Green Agenda Technology - Practices and Policies, Dubai, UAE, 8–10 December 2013.
[10]
EUROPEAN COMMISSION, “Ventilation units: Energy Savings”, retrieved from https://ec.europa.eu/info/energy-climate-change-environment/standards-tools-and-labels/products-labelling-rules-and-requirements/energy-label-and-ecodesign/energy-efficient-products/ventilation-units_en.
[11]
J. Al Dakheel, K. Tabet Aoul, “Building Applications, Opportunities and Challenges of Active Shading Systems: A State-of-the-Art Review, Energies, Vol. 10, No. 1672, retrieved from https://doi.org/10.3390/en10101672.
[12]
K. Cilento, “Al Bahar Towers Responsive Façade / Aedas E. Anderheggen”, ArchDaily, 5 September 2012, retrieved from https://www.archdaily.com/270592/al-bahar-towers-responsive-facade-aedas.
[13]
S. Attia, “Evaluation of Adaptative Facades: The Case Study of Al Bahr Towers in the UAE, QScience Connect: Shaping Qatar’s Sustainable Built Environment, Volume 2017, No. 2, Feb. 2018, retrieved from http://dx.doi.org/10.5339/conn...
[14]
T. Winstanley, “AD Classics: Institut du Monde Arabe / Enrique Jan – Jean Nouvel – Architecture Studio”, ArchDaily, 2 October 2011, retrieved from https://www.archdaily.com/1621...
[15]
E. Pelicaen, Development of an Adaptative Shading Device Using a Biomimetic Approach: Redesign of the South Façade of the Arab World Institute, Master Thesis, Brussels Faculty of Engineering, Vrije Universiteit Brussel (VUB 2018), retrieved from https://www.scriptieprijs.be/scriptie/2018/development-adaptive-shading-device-using-biomimetic-approach.
[16]
A. Petersen, “Mashrabiyya”, Dictionary of Islamic Architecture, Routledge, 1996.
[17]
Flectofold was first exhibited as part of the BauBionik Exhibition from 17.10.2017 to 01.05.2018 at the Natural History Museum in Stuttgart, Germany, retrieved from https://www.itke.uni-stuttgart.de/archives/portfolio-type/flectofold.
[18]
J. Lienhard, S. Schleicher, S. Poppinga, T. Masselter, M. Milwich, T. Speck and J. Knippers, “Flectofin: a Hingeless Flapping Mechanism Inspired by Nature”, Bioinspiration & Biomimetics, Volume 6, No. 4, Dec 2011, pp. 1-7, retrieved from http://iopscience.iop.org/1748-3190/6/4/045001.
[19]
“The Air Flow(er) is an energy independent thermally active ventilation device which behaves like a flower, whose “petals” open wide when exposed to warmer temperatures,” retrieved from http://www.liftarchitects.com/air-flower/.
[20]
A. Furuto, “Bloom / DO|SU Studio Architecture”, ArchDaily, 11 March 2012, retrieved from https://www.archdaily.com/215280/bloom-dosu-studio-architecture.
[21]
Achim Menges’s Meteorosensitive Pavilion belongs to the permanent collection of the FRAC Centre, based in Orléans (France), retrieved from http://www.achimmenges.net/?p=5612.
[22]
Ch. Darwin, The Power of Movement in Plants, D. Appleton and Company, New York, 1881, p. 317-417.
[23]
Q. Guo, E. Dai, X. Han, S. Xie, E. Chao and Z. Chen, “Fast nastic motion of plants and bioinspired structures”, Vol. 12, Journal of the Royal Society Interface, 6 September 2015, retrieved from http://doi.org/10.1098/rsif.2015.0598.
[24]
I. Burgert, P. Fratzl, “Actuation systems in plants as prototypes for bioinspired devices”, Philosophical Transactions of the Royal Society A: Mathematical, Physical and Engineering Sciences, 367, 28 April 2009, retrieved from http://doi.org/10.1098/rsta.2009.0003.
[25]
H. Modin, Adaptative Building Envelopes, Master Thesis, Dept. of Civil and Environmental Engineering, Chalmers University of Technology, Göteborg (Sweden), 2014, retrieved from http://publications.lib.chalmers.se/records/fulltext/214574/214574.pdf.
[26]
Engineered Material Solutions (Wickeder Group), Thermostatic Bimetal Designer’s Guide, retrieved from https://www.emsclad.com/fileadmin/Data/Divisions/EMS/Header/Bimetal_Desingers_Guide.pdf.
[27]
A. Y. Villarceau, Recherches sur le movement et la compensation des chronomètres, Mallet-Bachelier, Paris, 1862 [1st ed.].
[28]
W. G. van Doorn, Uulke van Meeteren, “Flower opening and closure: a review”, Journal of Experimental Botany, Vol. 54, No. 389, 1 August 2003, pp. 1801-1812, retrieved from https://doi.org/10.1093/jxb/erg213.
[29]
T. Nenov, “Development of adaptive shading device from thermostatic bimetal components using a biomimetic approach”, Biovoie Working Papers, 2018.
[30]
M. Schachinger, “Module Price Index”, PV Magazine, retrieved from https://www.pv-magazine.com/features/investors/module-price-index/.
[31]
D. L. Chandler, Explaining the plummeting cost of solar power, MIT News, 20 November 2018, retrieved from http://news.mit.edu/2018/explaining-dropping-solar-cost-1120.
[32]
M. Barozzi et al., “The sustainability of adaptive envelopes: Developments of kinetic architecture”, Procedia Engineering, Vol. 155, pp. 275-284, 2016, retrieved from https://doi.org/10.1016/j.proeng.2016.08.029.
[33]
INDUSTRY TODAY, “Solar Shading Systems Market 2017 Global Analysis by Key Players”, 25 October 2017, retrieved from https://industrytoday.co.uk/energy_and_environment/solar-shading-systems-market-2017-global-analysis-by-key-players---hunter-douglas--lutron--colt-international--rolashades--sunliving--novo-sunshading.
[34]
CIRC / IARC, “À propos du CIRC – Nouveau Centre”, retrieved from https://www.iarc.fr/fr/a-propo...
[35]
ArtBuild, “CIRC-IARC Lyon – International Agency for Research on Cancer”, retrieved from https://www.artbuild.eu/projec...
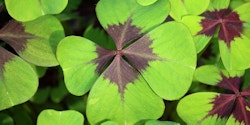